|
5G, the latest advancement in cellular network evolution, promises pioneering improvements in availability, reliability and performance requirements of the emerging applications in the enterprise segment.
Standard bodies like ITU and NGMN have come up with multiple use cases for 5G.
Each of these use cases differ in terms of expected data rates, latency, reliability and availability. Hence, they need different treatments by the underlying cellular networks.
This article discusses such use cases, shortcomings in 4G to realize these use cases and how 5G promises to handle their stringent requirements.
The article further attempts to show a high level migration from 4G EPC to 5G core network – mapping their network components with respect to functionalities.
5G Usage scenarios
Based on the requirements from the wireless networks, the 5G use cases are classified into three main categories:
- Enhanced Mobile Broadband (eMBB): These scenarios require 5G cellular networks to support very high data rates. 5G performance targets recommended by ITU suggest, peak data rate of 20 Gbps in downlink and 10 Gbps in uplink. In dense areas, it expects a throughput of 10-100 Mbps/m2.
- Ultra-Reliable and Low Latency Communication (URLLC): These scenarios require low latency and high reliability. 5G networks are expected to support <1 ms latency in access network and <10 ms latency end to end, for low-latency applications such as AR/VR, V2X communication, ehealth services. ITU further recommends a reliability > 99% (meaning that a packet reaches the destination in the allowed latency budget in 99% of the cases) and even close to 99.999% for specific deployment scenarios and use cases.
- Massive machine Type Communication (mMTC): In these scenarios, 5G is expected to support very high connection density. Smart cities, Industrial IoT cases fall under this category. They have large number of devices connected to the network – generally these applications expect low data rates. ITU recommended 5G targets expects handling between 10,000 and 1,000,000 devices per km².
4G shortfalls and how 5G helps
Performance requirements needed to satisfy the aforementioned scenarios can’t be fulfilled by the 4G networks:
- For 4G networks, ITU's International Mobile Telecommunications Advanced program indicates peak data rate of 1000 Mbps in downlink and 500 Mbps in uplink. This data rate is insufficient to support the eMBB scenarios. 5G networks are expected to use much wider frequency spectrum than 4G – with 5G NR mmWave running close to 30 GHz. Wider channel bandwidths and massive MIMO enable 5G to achieve data rates of 20 Gbps in downlink and 10 Gbps in uplink.
- Multiplayer gaming applications, industrial robots, self-driving cars and many such time-critical applications demand quick response – at times requiring network latency less than 1 ms, which 4G networks struggle to achieve or can't manage at all. 5G cellular technology is a key enabler to ‘edge computing’, thus bringing the compute power(applications) closer to the user and hence help reducing the network latency.
- Different network characteristics are required for each category of applications mentioned above. But it’s monetarily infeasible to have separate physical networks for each of them. Existing QoS solutions can classify and manage different types of IP traffic flowing over a given network but they are deficient in multiple ways. For instance, they can’t differentially treat same type of traffic from different tenants. At times, there is a need to provide mobile virtual network operators their own virtual infrastructures. In nutshell, there is a need to separate users, devices and applications that require a different quality of service for different use cases. This is where 5G network slicing offers cross-application, cross-service and cross-tenant segmentation of a network. It provides logical, self-contained networks comprising of a mixture of shared and dedicated resource instances - which includes network, compute, and storage capacity resources, virtualized network functions, and radio resources
Key principles of 5G
5G architecture is significantly different from its predecessors. This is crucial to meet the expected business and performance objectives, with respect to latency and reliability Following are some key principles that 5G networks will follow:
Separation of user plane and control plane: This separation will enable independent scaling and flexible deployments for each of the planes. Resource assignments can be done based on needs. For instance, applications needing high bandwidth, like video surveillance, can scale user plane functions. Whereas, IoT application which require few bytes of data exchange but more frequently, might require scaling of control plane functions more frequently. This separation also allows independent movement of some functions closer to the user while keeping others centralized in the cloud.
Services based architecture (SBA) for network functions: 5G core network functions use services-based interfaces for their interactions. Each of these network functions provide services to another network function. A service consists of operations, based on either a request-response or a subscribe-notify model. With SBA, same operations can be reused by other existing functions or new functions.
Stateless network functions: 5G network functions are designed to be stateless. Separating state from the control, allows control to run on a compute resource and state can be saved at a separate storage node. Apart from enhancing reliability by maintaining redundancy of storage nodes, this statelessness also aids in dynamic instantiation/scaling of virtual network functions corresponding to the 5G network functions.
Support for capability exposure: 5G allows external functions to interact with their core network functions using ‘capability exposure’ function. This function allows the external functions to retrieve state/status/events from the 5G core and to pass configurations/policies to the 5G core. This 5G feature is a key enabler for multi-access edge computing paradigm.
Local and centralized access: To handle the mission-critical and low latency applications, 5G allows one PDU session to have multiple user plane anchors towards the data networks. This lets 5G support concurrent access through local compute servers (at edge) as well as centralized cloud servers. Compute capability at the edge help in reducing the network latency enormously.
Introducing 5G
5G relies heavily on virtualization – it plays a key role in the flexibility and scalability requirements that 5G expects. It allows movement to vendor-agnostic implementations and efficient sharing of hardware.
At the core side, 5G is a collection of network functions, expected to be virtualized and run on a cloud infrastructure. In transition from 4G to 5G, 4G EPC functions have been modularized to form multiple 5G core network functions, thus re-architecting them as cloud-native applications. Following are some examples of the partitioning done:
- The 4G Mobility management entity (MME) functionality has been divided into 5G Access and Mobility management Function (AMF), session management function (SMF) and Authentication Server Function (AUSF):
- AMF handles the UE registration and mobility management
- SMF handles the PDU session management
- AUSF handles the UE authentication part based on authentication vectors from Unified Data Management (which stores the UE subscription data and user-context).
- The 4G S-GW and P-GW have been divided into session management function (SMF) and user plane function (UPF):
- SMF handles the UE IP address assignment and UPF selection
- UPF acts as PDU session anchor – it is responsible for packet routing, QoS enforcement and charging functionality
The diagram underneath gives a high level mapping of 4G EPC functions to 5G core network functions.
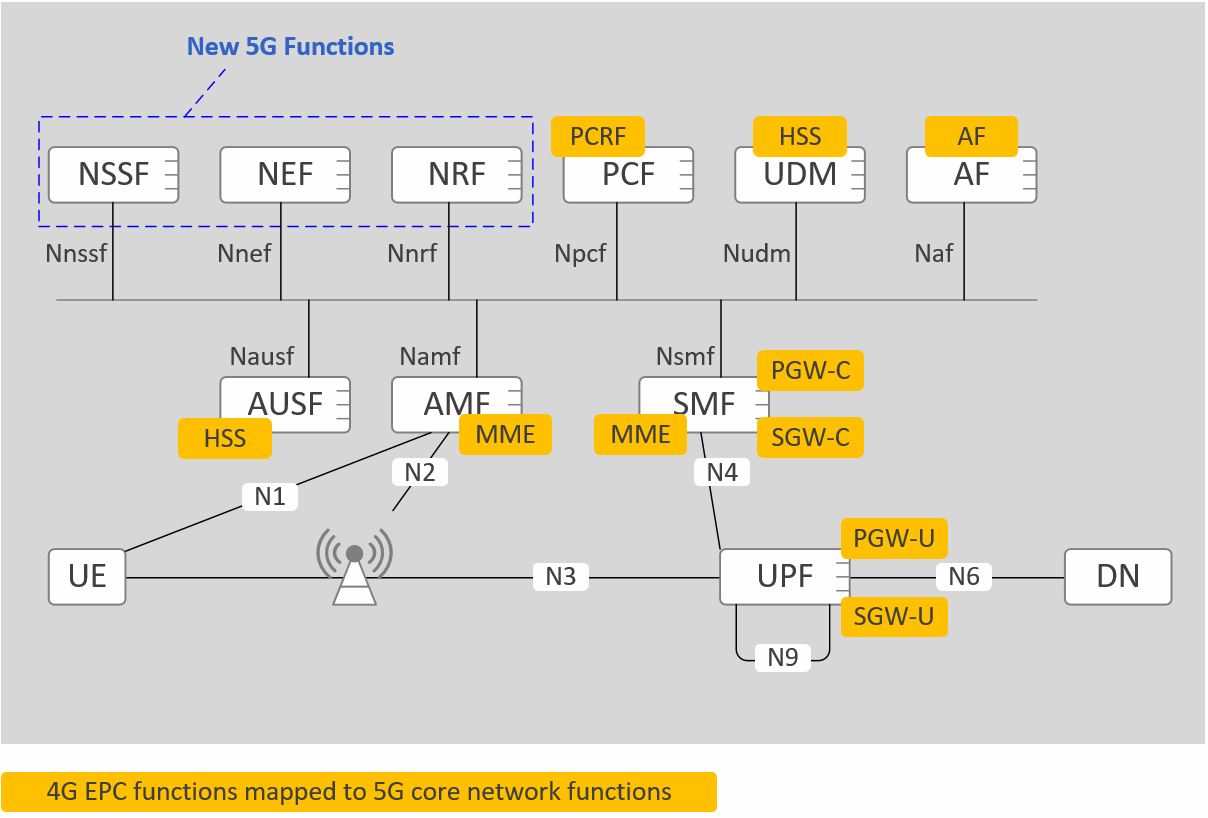
Figure 1: 4G EPC vs 5G Core
Following are the new 5G core functions introduced:
- Network Slice Selection Function (NSSF): NSSF helps in setting up multiple virtual network slices of the RAN, core and transport networks to meet specific service requirements.
- Network Exposure Function (NEF): This is the capability exposure function used by the external network entities to interface with 5G core network.
- Network Repository Function (NRF): It aids the 5G services based architecture. It acts as repository of all services offered by the different network functions.
In order to support high data rates and low latency needs of 5G, the access network and fronthaul have been revamped. At the access side, the 4G eNodeB (eNB) is now called 5G gNB (next-generation node B):
- The gNB is split into two parts – gNB centralized unit (gNB-CU) and gNB distributed unit (gNB-DU). Both are connected to each other using ethernet based IP midhaul network.
- Generally, gNB-CU is virtualized and runs in context of virtualized core or virtualized edge.
- gNB-DU might be integrated with the radio head or can be virtualized and run on the edge or cloud.
Conclusion
5G is opening up a new business opportunities from diverse market segments like healthcare, smart cities, connected devices, industrial IoT etc. Each of the 5G use cases differ in terms of requirements with respect to data rates, latency, connection density and reliability.
Virtualized access and core networks, modularized network functions and varied deployment options of 5G will actively help in realization of apt cellular connectivity for these diversified markets.
|
|